Written by Karsen Kitchen, Ishaan Balakrishnan, Jacob Blizzard, James Bowling, and Rianne Eccleston.
Module 3 centered on the exploration of star birth regions, with our particular emphasis on the Tarantula Nebula, where we investigated the physical mechanisms involved in the formation of new stars. In contrast, Module 5A is dedicated to the study of regions where stars die, with a focus on analyzing the differences in chemical composition and physical processes that occur in these areas. As part of this module, we were tasked with selecting a star death region to study and analyze using data obtained from the afterglow image editing software. After careful consideration, our group chose to focus on the planetary nebula known as “Spiral,” which is also designated as NGC 5189. Through our analysis, we aimed to gain insight into the physical and chemical processes at play in this fascinating region of the universe. A planetary nebula is formed when a low-mass star, such as our Sun, runs out of fuel and begins to shed its outer layers. This process creates a shell of ionized gas and dust that expands outward, forming a nebula. Planetary nebulae are typically short-lived, lasting only a few tens of thousands of years before they dissipate. A protoplanetary nebula, on the other hand, is a short-lived transitional phase that occurs before a planetary nebula forms. During this phase, a dying star sheds its outer layers and creates a shell of gas and dust around it. The star’s core, which will eventually become a white dwarf, is still visible at the center of the shell. Protoplanetary nebulae are characterized by their complex and often asymmetric shapes.
To start, we set our target, and updated our coordinates with our preferred coordinates. We set our Max Sun Elevation to -18 degrees, Min Target Elevation to 30 degrees, Min Visible hours to 1, Max Moon Phase to 90%, and our Min Moon Seperation to 60 degrees. Then, we selected prompt 5 as our telescope, since the spiral nebula is quite small. For our filters, we are only selecting Halpha and OIII, since our nebula is so far away, and then set our exposures. For Halpha, we took one image and set the time duration to 300 seconds. For OIII, we also only took one image and set our time duration to 300 seconds as well. We want to repeat this observation 5 times with at least 30 minutes in betwen each.
Once our observations came back, it was time to insert them into the Afterglow editing software, to attempt to accurately determine the chemical composition of the Spiral nebula. While the star forming regions observed in Module 3 focused on many stars as well as the surrounding gas, star death regions (SDR’s) are slightly different. In these SDR’s, there is only really one star that is of importance. This is the ABG star for protoplanetary nebulae, the white dwarf star for planetary nebulae, and the WR star for Wind-blown bubbles. SDR’s, consequently, are much more focused on the surrounding gas. Because of this, the narrowband filter images that we captured will be utilized more heavily.
To highlight the presence of the gas in the NGC 5189 nebulae, we first uploaded our returned images into the Afterglow editing software. From there, we aligned, stacked and grouped our images, much like in previous modules, to achieve one coherent image incorporating each filter we obtained. Thanks to the development of the Afterglow software, there exists many differing color pallets that could be mixed and matched to most adequately highlight the gas. It is important to note that, in highlighting the gas to a sufficient degree, some color pallets were used that do not yield colors that are indicative of the true gas composition that is present. For example, the SHO color pallet, one that has been recently utilized to create some HUBBLE images, employs a traditional RGB (red, green, blue) color scheme. In this scheme, Hɑ is colored green, when the balmer color, a pink-red hue, is more true to the actual color of the ɑ-Hydrogen composition.
For our image, two distinct color pallets were chosen, and are displayed in the images below. The first image was colored with a Harmonized SHO color scheme. This pallet uses the colors OIII and Harmony (a deep blue color) for our Hɑ and OIII filters, respectively. Because we only obtained Hɑ and OIII narrowband images, the SII filter and color combinations will be omitted from this discussion. The second image was colored with a HSO color scheme, which differed from the first by utilizing Red and Blue to achieve the more purple hue shown. In each image, the mixing of the two filter colors is indicative of concentrations of both Hɑ and OIII gasses.
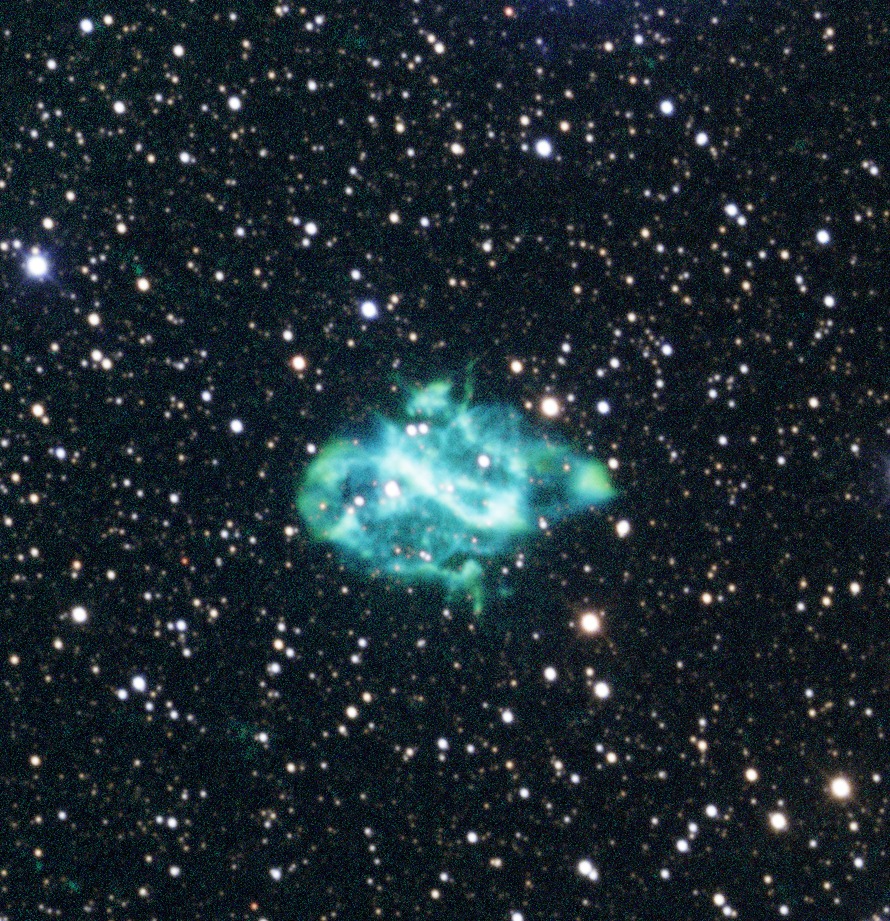

After initial processing comes the addition of archival data. This archival data usually consists of dust not seen by Prompt telescopes, instead from more powerful telescopes used by NASA. To get these pictures it needs to be retrieved from different websites depending on which archival data is to be used. WISE 12, WISE 22, and 2MASS data can be retrieved from the same website: NASA’s SkyView. Spitzer data needs to be taken from a different CalTech website: IRSA, a combination between NASA and IPAC. Spitzer can be seen as a newer version of WISE data, 2MASS will not be used in this case as it doesn’t focus on dust itself.
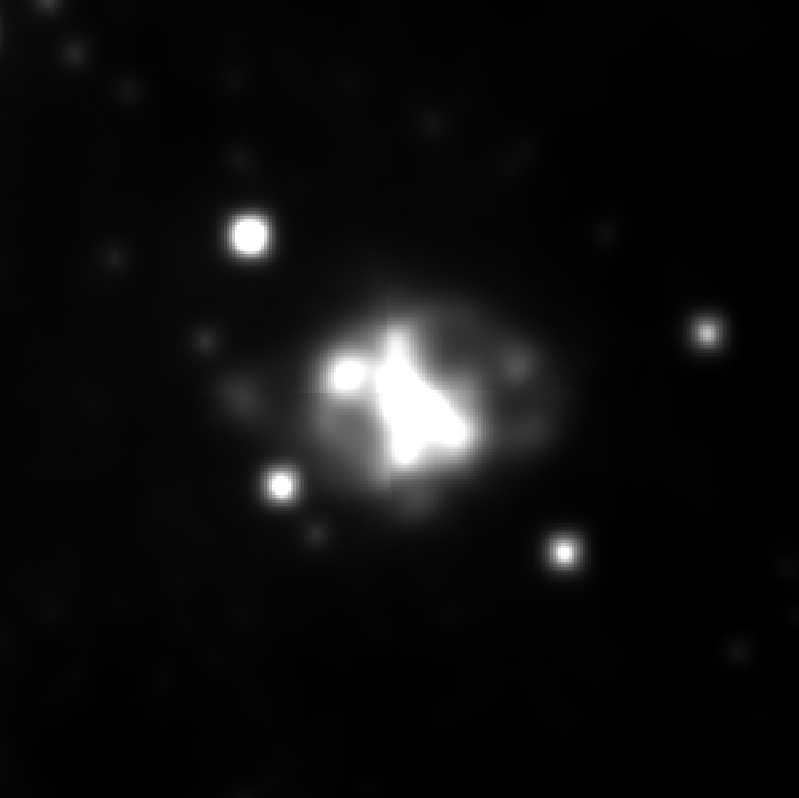
(Example dust data from WISE 22)
The archival data used for NGC 5189 is WISE 12 and 22, 2MASS wasn’t used and there was no Spitzer data for the star death region. Adding the two WISE data files into afterglow isn’t hard once it’s downloaded. Since it’s archival there’s no need to use cosmic correction or other algorithms to clean the image. Instead it’s straight to aligning, since these are all different regions it’s best to align all of the stacks in the group plus the archival data to one of the pre-existing stacks in the group, I chose Halpha. There is also no need to stack the images as well, as it is one image there is nothing to stack. Grouping them is similar to narrowband images, going under narrowband and above luminance. Then it’s on to choosing the colors for this dust data and adjusting the saturation level, I chose to use a cool color map on WISE 12, and a hot color map for WISE 22. I used a HOO color scheme for my picture as there was no SII filter to color, so OIII took on a blue-green color map, while Halpha used balmer, closest to its’ natural color. All in all, it resulted in this picture:
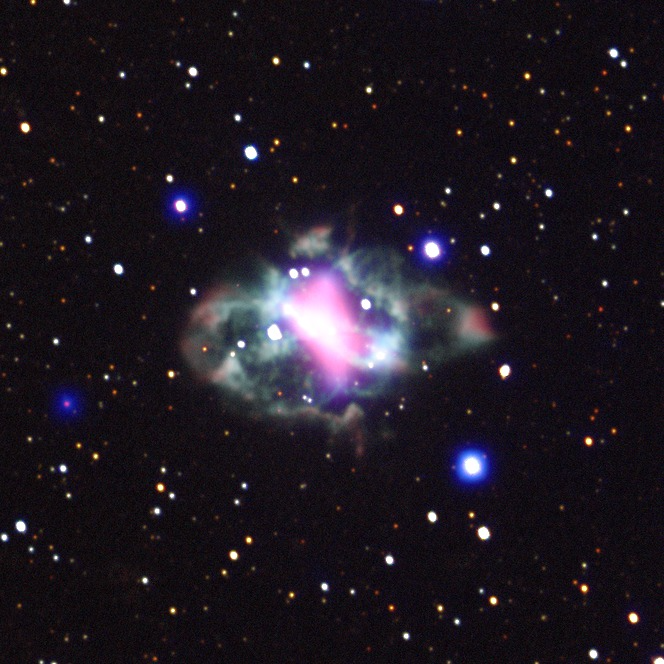
The different colors captured in the planetary nebula give insight into the physical processes occurring. We can learn about the temperature distribution given the different color maps applied to the filters. OIII is typically in the hottest region, with a temperature of up to 40,000k and visualized in the blue-green wavelength. H-alpha appears pink, with temperatures ranging from 5,000k to 10,000k. Archival data from Spitzer and Wise are the coolest regions, and typically further away from the center star. We can also learn about the density of the nebula through the structural filaments that form. These can be seen throughout our planetary nebula, spiraling through the center and particularly dense at the edges. These are created when there are instabilities that cause cooler, high-density gas to become engulfed with hotter, lower-density gas. They can also be shaped by ionizing radiation and stellar winds, indicating pockets of higher density. The nebula is particularly dense on the outer regions due to the star ejecting gas out as it dies. As the gas begins to slow down, it piles up at the edges and creates regions of higher density.
NGC 5189 is a planetary Nebula located in the constellation Musca. This nebula was formed by the outer layers of a dying star that ionized by the intense radiation of the central star. Ionization, excitation, and radiation are some of the physical processes at work in NGC 5189. Ionization is the first process at work in NGC 5189. The center star’s powerful UV emissions ionize the nebula’s gas, removing the electrons from the atoms and leaving behind positively charged ions. The intensely colored nebula is produced by highly charged ions that generate light when they recombine with electrons. Excitation is the second process. Light is produced as a result of the nebula’s electrons and ions recombining. Not all of this energy, though, is emitted as light that can be seen. A portion of the energy is released as UV radiation, which has the ability to ionize nearby atoms and molecules and restart the process by ionizing them. Radiation pressure is the final factor. The nebula’s gas is under pressure from the central star’s powerful radiation, which causes the gas to expand and give the nebula its characteristic structure. The original star’s outer layers, which were ejected into space and molded by the star’s own rotation and magnetic field, also had an impact on the shape of NGC 5189. Overall, the surrounding gas is ionized by the intense ultraviolet radiation that the core star, a white dwarf, generates. This then causes the gas to become charged as a result, which in turn causes it to emit light. Then, the color and shape of NGC 5189 are due to the light that is emitted from ionized plasma.