Written by Karsen Kitchen, Ishaan Balakrishnan, Jacob Blizzard, James Bowling, and Rianne Eccleston.
In module 2, we studied star clusters which consist of groups of stars that are born together. We learned how to measure the brightness and apparent colors of stars, which in turn can help us determine their distance and age. We examined three star clusters of varying ages: young, intermediate, and old (globular). By gathering data using Skynet, we measured the age and metallicity of these clusters. To further analyze this data, we constructed an HR Diagram which allows us to visualize the distribution of luminosity and intrinsic colors of stars, providing insight into how the clusters evolve over time. Additionally, by measuring the metallicity of gasses emitted by the stars in the clusters, we can gain a better understanding of their chemical composition. HR Diagrams are an invaluable tool for studying stellar evolution and the behavior of star clusters.
In each group, we observed 3 separate star clusters. Our group observed NGC 2910, our young cluster, NGC 1245, our intermediate cluster, and NGC 4833, our globular cluster. In Skynet, we added a new observation and looked up each of our targets. We set our Max Sun Elevation to -18, Minimum Target elevation to 20, Minimum Visible hours to 1, Maximum Moon phase to 20, and Minimum Moon separation to 60. For our filters, we selected the B,V,R, and I filters. Since we are observing these clusters more for the data behind them rather than the aesthetic, we chose all the PROMPT telescopes instead of just one. We then selected the Generic 16-Inch telescope and set our exposures. We took one exposure for each filter and set our durations to 30.0, 20.0, 10.0, and 15.0 for each respective filter. We repeated our observation 5 times with at least 30 minutes between images to avoid ghosts.
So now that we have all of our pictures back from observations, we start to process them in Afterglow to have them show their initial colors. First, like most other observations, is the stacking and the aligning of the images. First we clean the images, as they aren’t perfect yet, at the time of the initial image editing, we had to run a long string of a command, especially if pictures were taken on Prompt-6.
Aligning them comes with ease as the pictures were automatically given their coordinates in right ascension and declination, so Afterglow knows where you are in the sky based on the picture’s coordinates. Aligning it was as simple as Afterglowing taking a reference image’s world coordinate system and then applying them to the rest of the images, then cropping them to get them all to the same size. The best reference image to use is taken in red, or R, as it captures the most light from stars compared to the other three filters.
(ngc 2910)
Stacking gets a little different this time around, but not by much. The deeper into space one takes pictures of, the easier it is to not notice bad pixels or anything that wasn’t cleaned up from the start. With the normal stacking procedures of doing it by filter, we can add rejection to get rid of these bad pixels. Called “chauvenet,” the stacking program removes all outliers by iteration, essentially sigma clipping. Setting the low and high settings to 1 gives the desired effects.
(rejection on left, both in r filter, ngc 1245)
Now that the pictures are aligned, stacked, cleaned, and grouped together, it’s time to color them, except for I. I doesn’t affect the colors, just adds to the data that’ll be used for graphs later on. Coloring the stacks is similar to how it’s done for the planets, using the brightness of the background stars. It’s called photometric color calibration, used on the composite of the color maps when applied to the filters. This is under histogram fitting and is used instead of percentile because the stars aren’t white. It brings up a pop up which zero-points in the filters are used, along with blackbody radiation in the b filter to adjust the colors, which can then be adjusted manually after choosing a reference image. There was another option in that pop-up named extinction E(B-V), which is the amount of reddening in the picture because of the atmosphere. Because we don’t know this value yet, we can’t adjust the reddening, making the pictures much warmer than they should be. Once adjusting the brightness using midtone, we get our initial pictures.
While the pictures are done, there is still a reddening problem that can be fixed, but more information can be extracted out of these pictures, like age. This is done using photometry, which measures the brightness of each star in the cluster, automatically, through batch photometry. This is so we can do it on a number of targets that would be too many to do by hand, often in the thousands. Using the APASS catalog, a sky survey with calibrated stars in multiple filters, we use that in conjunction with the star clusters’ WCS to figure out which stars overlap using the red filter. This is then sourced into all other pictures of the stack, which can be used to then extract these sources from the whole image. But as this only does one stack at a time, we can do all four with ease by using a different tab on Afterglow, the photometry tab. This takes the sources and injects them into the four stacks, creating a spreadsheet for each star in each filter. Creating these spreadsheets takes a while, as it has to see if each star is in APASS, and then measure the brightness for thousands of targets, four times with the filters, and then 12 times by having the three overall clusters. These spreadsheets are readable by us, but it’s better used in the graphing sequences so that the brightnesses of the stars in the clusters can be seen visually, we can also get our reddening value from this, and see the true colors of our clusters.
(spreadsheet of ngc 1245 right, source extraction for photometry left, ngc 2910)
To create an HR Diagram for our data we need to start by downloading the batch photometry data from afterglow after processing our images. We will then upload this data into the graphing tool called “cluster pro plus”. We then plot our B-R on the x axis and the V data on the y axis for the first diagram. Then we plot the V – I data on the x axis and the R data on the y axis for our second diagram. Then we need to remove the dimming and reddening effects of the intervening dust for which we increase the E(B-V) slider. Then we use the measurements from the GAIA spacecraft for the parallax and how quickly each star is moving across the sky, in both RA and Dec. We then use the “Motion in RA” and “Motion in Dec” sliders on the left which are measured in milli-arcseconds per year making the GAIA very sensitive. We then change the range below which helps clean the diagrams a lot by cropping the field stars. Finally we find the distance to the clusters to crop out any stars that are not in this distance. The default 30% works pretty well for this as it takes in any GAIA uncertainties. Then you find the distance which is halfway between both distances to get your final HR Diagram for your star clusters.
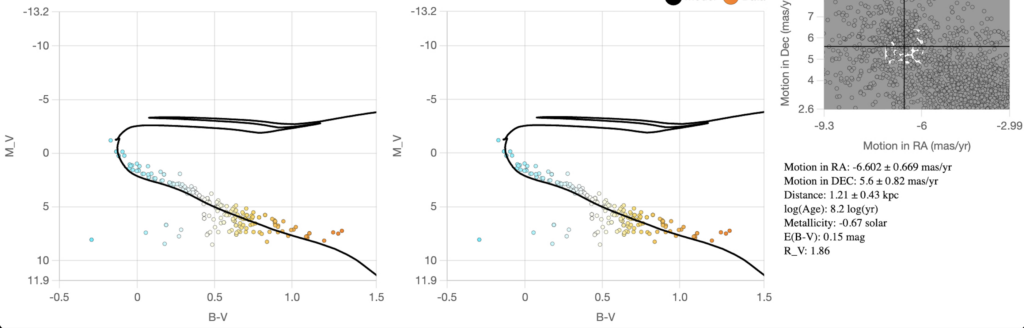
(Graph of NGC 2910)
This is our HR Diagram of our young cluster, NGC 2910. From our HR Diagram for this open cluster we can understand that our original analysis gives this cluster an age of log(age): 8.71. Along with this the original metallicity is shown to be -0.03.
(Graph of NGC 1245)
This is our HR Diagram of our intermediate cluster, NGC 1245. From our original HR DIagram for this open cluster we found the age to be around log(year) = 9.39 but after using our comparison graphs for both the NGC 2910 and the NGC 1245, we found our intermediate cluster to indeed being older than our young cluster, NGC 2910. This is because 8.71 log(year) is less than 9.39 log(year). Along with this we also found the metallicity for this open cluster to be really low at -1.11 which is typical of older open clusters.
(Graph of NGC 4833)
This is our HR Diagram of our old globular cluster, NGC 4833. From our original HR Diagram for this globular cluster, we found the age to be around log(year) = 10.2 which, after using the comparison graphs, stayed the same. We also found the metallicity of this globular cluster to be around -1.97, which makes sense as the oldest star cluster to have the most pristine gas out of the rest of our star cluster observations.
While we obtained a decently fit isochrone for each of our 3 star clusters, it was possible to further refine our analysis by including archival information from the sources GAIA and 2MASS. The GAIA data came from a 20-inch diameter equivalent telescope in space, while the 2MASS data originates from two 1.3meter diameter telescopes on the ground. Because GAIA is in outer space, it is able to image regions of interest with much less interference. Including this data will thus refine our isochrones by giving a more accurate representation of the stars present in that area. GAIA images were taken using the filters; BP (blue), G (green), and RP (red). 2MASS, on the other hand, is a NIR (near infrared) survey of the sky from Earth’s surface, and its data was taken using the filters; J (bluer), H, and K (redder). All 3 of these filters are redder than the standard BVRI filters used for our imaging, and there is good reason for this. Due to these redder wavelength filters, dust interference is much less common.
By including these two data sources and refitting our isochrones for each star cluster, the group was able to achieve a much more accurate fit. The young cluster, NGC 2910, saw a decrease in age by 0.218, which corresponds to being roughly 70 million years younger than we originally postulated. Along with that, the metallicity increased from -0.14 to -0.04, and the reddening factor decreased from 0.393 to 0.227. A metallicity decrease to almost 0 indicates that the mixture of elements in the gas that formed this star cluster is very similar to that of our sun. This makes sense, because our solar system is extremely young on a cosmic timeline. Younger clusters have been enriched with remnants of other supernovae and thus contain much more elements than older clusters, which were formed with relatively pristine gas and thus have lower metallicity values. This becomes apparent when observing how the metallicity values for our intermediate and old globular clusters become increasingly negative. Furthermore, a decrease in the reddening indicates that there is not as much interference between us and our target region.
Next to refit was the intermediately aged cluster, NGC 1245. Being the intermediate cluster, it was assumed that this cluster would be older than the young cluster NGC 2910. This was proven analytically, with an original age of log()=9.2, and an archive-assisted age of log()=9.08. This difference corresponds to the intermediate cluster being younger than originally analyzed by about 380 million years! It seems impossible, but for these star clusters with ages in the multi-billions of years old, this is a natural timeframe. Along with the age, the metallicity decreased from -0.71 to -0.26, again indicating that the elements in the gas of the cluster’s formation is somewhat relatable to our solar system. Finally, our isochrone for NGC 1245 had the same value of reddening in both our original data as well as the archival-included data.
Finally, our isochrone for our old globular cluster NGC 4833 was refitted. This is the oldest cluster, and thus should possess the oldest age. It does indeed, with the archival data matching the original data with a log() age = 10.2. It should be noted that the maximum log(yr), 1010.2 = ~15 billion years old, is approximately the age of the universe. Thus, the NGC 4833 cluster cannot be older than log()=10.2. however the isochrone model in Skynet that we utilized only fits to a maximum log() = 10.2 age. Because of this, it can be reasonably stated that there is some external factor affecting the fit of the isochrone, causing it to appear older than it actually is. This can be attributed to multiple potential reasons. For one, the cluster itself may have formed at different times. Multiple formation periods, and the fact that dust from each formation period might not have mixed fully, can cause there to be multiple metallicities. The presence of multiple metallicities may have thrown off the isochrone fitting, giving the overestimated age seen in the graph below. The metallicity of NGC 4833 decreased from a value of -1.97 originally to a value of -2.05. This makes sense, as the oldest cluster would have the most pristine gas and, thus, the lowest presence of extraneous elements. The reddening for NGC 4833 stayed relatively the same, decreasing from 0.33 to 0.32. The globular cluster ended up being the easiest cluster to fit to an isochrone, because it has many more distinct stars than the other 2 clusters.
Once we determined how much dust is in between the Earth and each of the star clusters, we adjusted the redness of the stars to determine the true intrinsic colors. Using the photometric color calibration in Afterglow one more time, we recalibrated the colors of our clusters to account for the blue wavelengths being blocked by dust. Once we subtracted the redness out, we were left with images of star-forming regions with colors as they would appear with the naked eye, if only we were much closer. Now that we had our true colored image, the only thing left to do was test our intuition in determining the ages of the clusters based on their intrinsic colors alone. The globular cluster is always the easiest to identify, as its dense spheroidal shape is unique to these ancient clusters. Formed after the collapse of massive dust clouds throughout the universe, globular clusters contain some of the oldest stars. Because they were formed so long ago, they contain noticeably more red stars than any of the other clusters. In the Milky Way Galaxy, the globular clusters have an average age of around 14 billion years old. The ages of the open clusters can be more difficult to identify, as their features are less distinguishable than those of the globular clusters. Both of the clusters are significantly bluer and more spread out, so it is hard to date them based on images alone. However, since the intermediate clusters tend to contain older stars, they generally appear just slightly redder than the younger clusters, giving the indication that they may be slightly older. Our team took a stab at dating another set of clusters, and successfully ranked all three of them in age order.
After collecting this data and analyzing it through various methods, we can infer that the study of star clusters provides us with valuable insights into the evolution of stars and the structure of the universe. Through the use of Skynet to gather data and then using afterglow to process the images and colorize it, we were able to measure the brightness and colors of stars in clusters and determine the distance and age of these clusters. We were also able to create HR diagrams using the data from our images to visualize the evolution of the clusters over time, as the diagrams allow us to visualize the distribution of luminosity and intrinsic colors of stars. Through photometry, we were able to measure the brightness of thousands of stars in different filters. We were also able to measure the metallicity of the gasses emitted by the star cluster to gain a better understanding of their chemical compositions. We worked as a team to choose different star clusters, put in the observations, and then work together to process these images and data to be able to analyze these images that we were able to capture.
To the left we can see our young cluster which is NGC 2910. Through our analysis in the HR Diagram we were able to understand that this star cluster had a decrease in age by about 0.218, which is about 70 million years less than we had originally analyzed it to be. Our young cluster is an open cluster in the Vela constellation, which is in the south of the celestial equator, therefore, making it more easily viewable from the southern hemisphere. Since we selected all PROMPT telescopes in Chile to get our observations back as fast as possible, we were able to take advantage of that.
In the middle we can see our intermediate-aged cluster, which is NGC 1245. This cluster was originally presumed to be older than NGC 2910, our young cluster. We were then able to compare the original age and the archive-assisted age which came out to show that the star cluster was 380 million years younger than the original age. This star cluster is in the constellation Perseus and was discovered by William Herschel on the 11th of December, 1786. This cluster is estimated to be about 27 light-years across, and it lies north of the celestial equator.
To the right we can see our old cluster, which is NGC 4833. By comparing our archival data and original data, we were able to find the age of this star cluster to be around log() age = 10.2, which is around 15 billion years old, which is a lot older compared to our intermediate, which is only around 1 billion years old. This is a globular cluster inside the Musca constellation, and it lies south of the celestial equator.
These are our three beautiful star clusters and thank you for reading!