Left to right – NGC 3293, NGC 3330 and NGC 4833; all images are 20 exposures taken on January 27, 2023 at various times; exposures for NGC 3293 and NGC 3330 were taken by PROMPT6 optical telescope in Cerro Tololo Inter-American Observatory, Chile; exposures for NGC 4833 were taken by PROMPT5 and PROMPT6 optical telescopes in Cerro Tololo Inter-American Observatory, Chile and by PROMPT-MO-1 optical telescope in Meckering, Australia. (The above images are stylized for effect, please see the images below for their unaltered versions.)
This article was composed in partner with Andreas Buzan, Alyssa Manus, and Ruby McGhee. Please see the credits for more information.
About the Images
Overview
For NGC 3293, the young cluster, we used the telescope PROMPT 6. We took a total of 5 exposures per filter with 30-minutes delays between each set of B, V, R, and I filters. The total observing time for each filter are as follows: B = 31.5 seconds, V = 23.6 seconds, R = 11.8 seconds, and I = 23.6 seconds. The reasoning for spreading out our exposure times is to a) prevent “ghost” images of brighter objects that may be captured in the observation and b) allow other classmates time to collect their data as well!
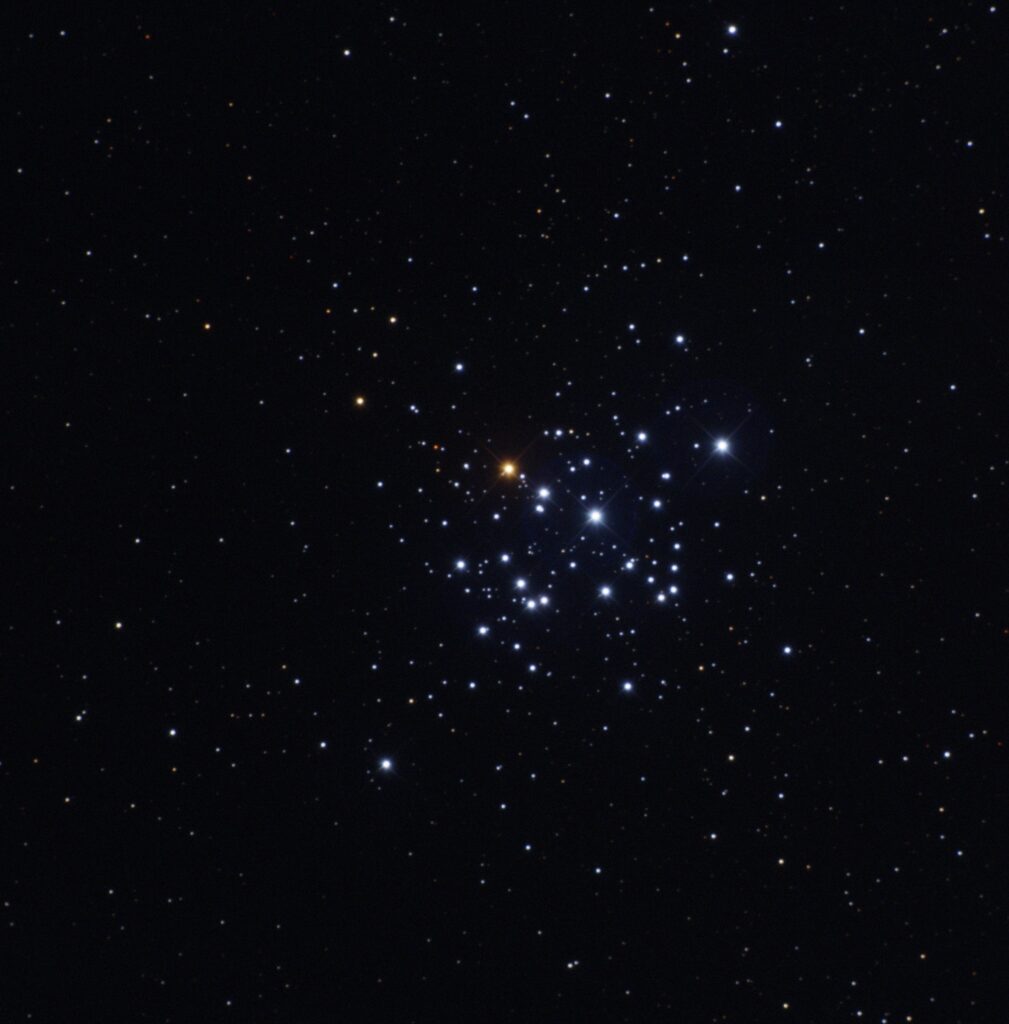
For NGC 3330, the intermediate cluster, we used the telescope PROMPT 6. We took a total of 5 exposures per filter with 30-minutes delays between each set of B, V, R, and I filters. The total observing time for each filter are as follows: B = 118.1 seconds, V = 59.05 seconds, R = 78.75 seconds, and I = 59.05 seconds. The exposure lengths for each filter vary because we need to collect quality data that consists of just enough time to capture the cluster’s dimmer stars without overexposing the brighter stars. This allows us to collect the unique photometric measurements through the wide array of magnitudes that each cluster contains.
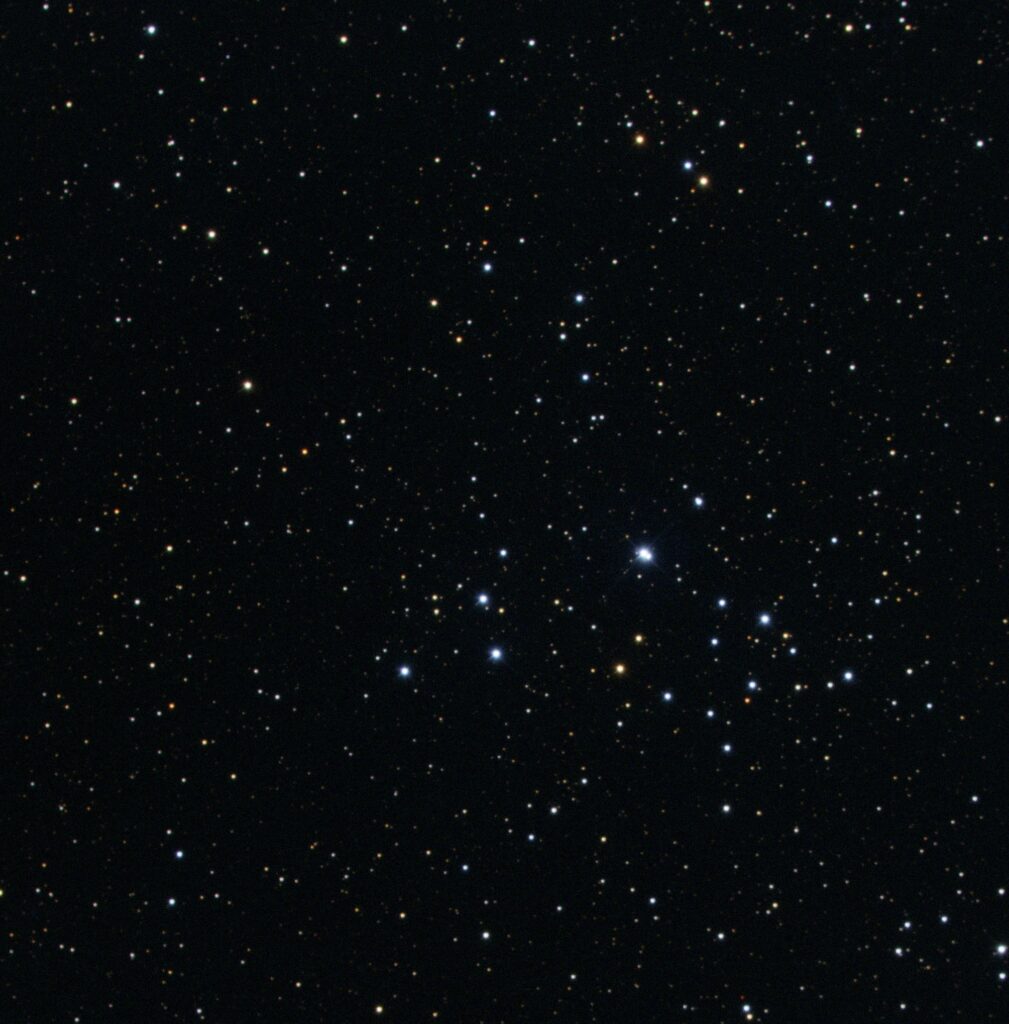
For NGC 4833, the globular cluster, we used the telescopes PROMPT 5, PROMPT 6 and PROMPT-MO-1. We took a total of 5 exposures per filter with 30-minutes delays between each set of B, V, R, and I filters. The total observing time for each observation are as follows: Prompt 5: B = 176.48 seconds, Prompt 5: V = 176.46 seconds, Prompt 5: R = 176.46 seconds, Prompt 5: I = 78.44 seconds, Prompt-MO-1: I = 51.95 seconds, Prompt 6: B = 141.74 seconds, Prompt 6: V = 94.48 seconds, Prompt 6: R = 94.48 seconds, Prompt 6: I = 62.5 seconds.
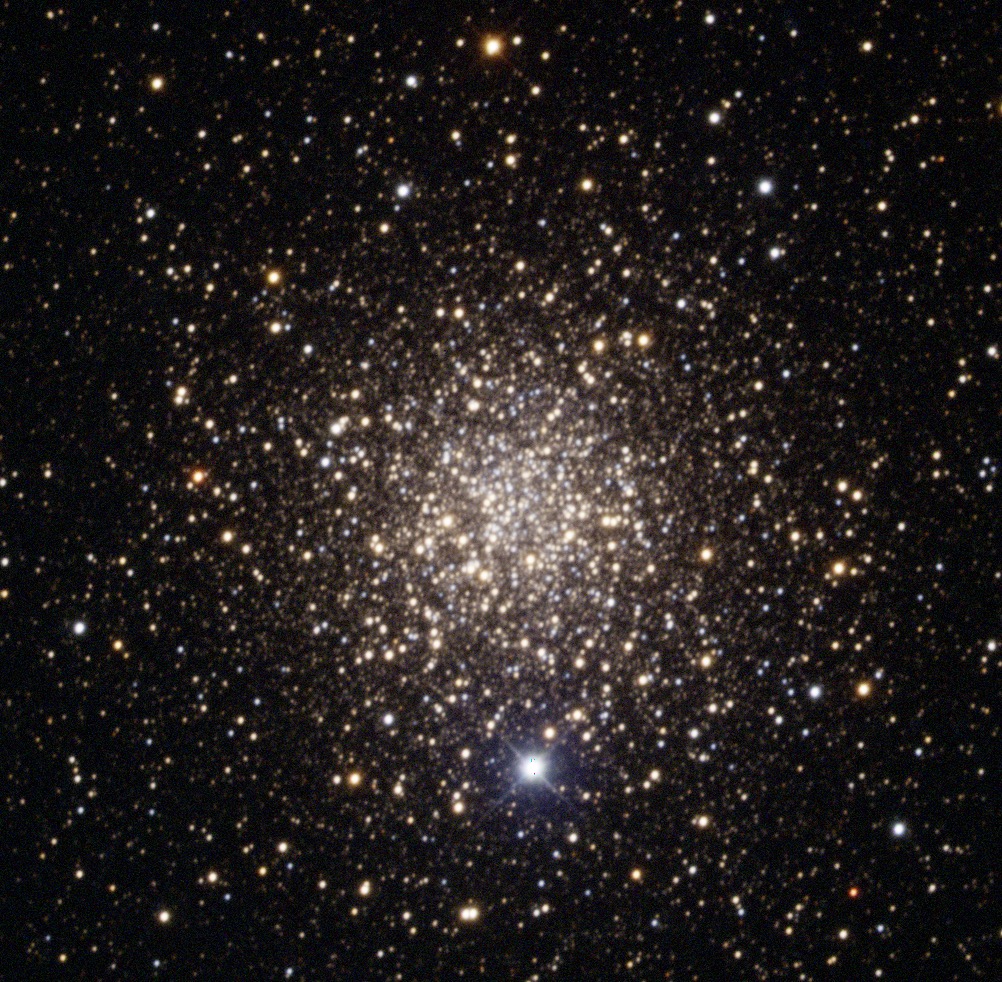
Color Calibrated Clusters
For each of these star forming regions, we aligned, stacked, and color balanced them the same way:
We first loaded all twenty exposures from the observation into Afterglow. Then, using the align tool, we selected all twenty images and used WCS Calibration to align them. Next, we selected the five exposures from each of the four filters (I, V, B, R) and used the stack tool with Chauvenet rejection and propagation mask enabled. With the four total stacks, we group them into one file and change the color map to its respective filter.
Now we have a full color image. To make the colors in the image more accurate, we used the histogram fitting tool. With photometric calibration open, we measure the zero points and select our reference layer. For all three of our clusters, we used the B filter layer as our reference layer. With that, we have a full color calibrated image!
HR Diagrams
How they’re made
After creating the colored images, we can use the batch photometry tool in Afterglow to create a CSV file measuring all the brightnesses of each star in the red, green, and blue wavelengths of the images. We loaded those CSV files for each cluster into the Skynet graphing tool in Cluster Pro Plus mode to generate a diagram that shows the ages of the individual stars in each cluster (an HR diagram). Using the option in the top right of the graphing tool, we can narrow down the stars that are shown in the diagram to just the ones in the cluster, completely ignoring the field stars. We can use this data to estimate the age, solar metallicity – how much metal (anything that isn’t hydrogen or helium) is in the stars on average, and reddening – how much dust is between us and the cluster – of each image. We also used data from the space telescopes GAIA and 2MASS to get the most accurate measurements possible of all the stars in each cluster. We then tweaked the values of age, metallicity, and reddening until the black line on the graph – the “turnoff” point, or the description of how stars change over time for stars in that cluster – lined up with the stars in our diagrams. We then compiled the data into 3 separate graphs shown below. The diagrams on the left shows how the stars change as they age in our visible wavelength (B, V, R), while the diagrams on the right shows how they change in the near-to-mid infrared wavelengths (J, H, K).
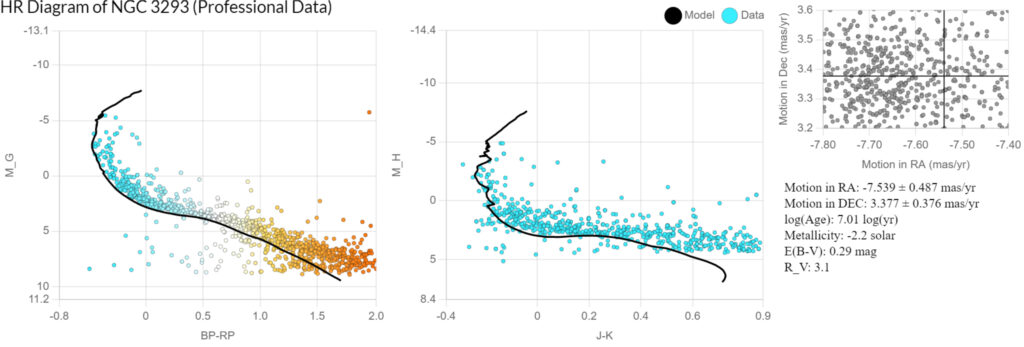
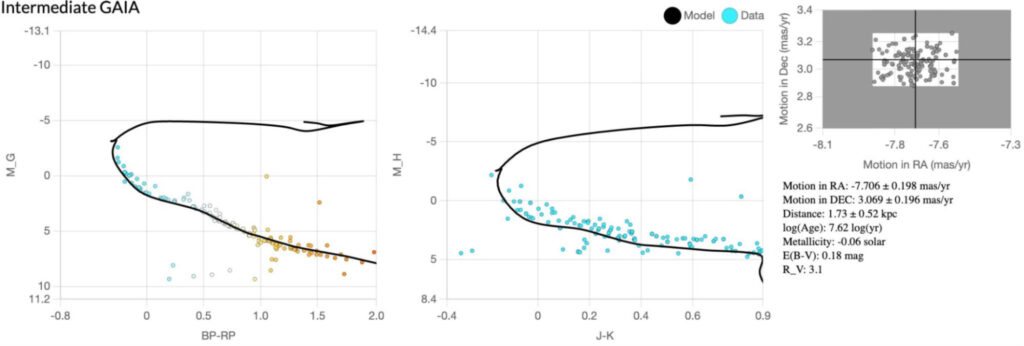
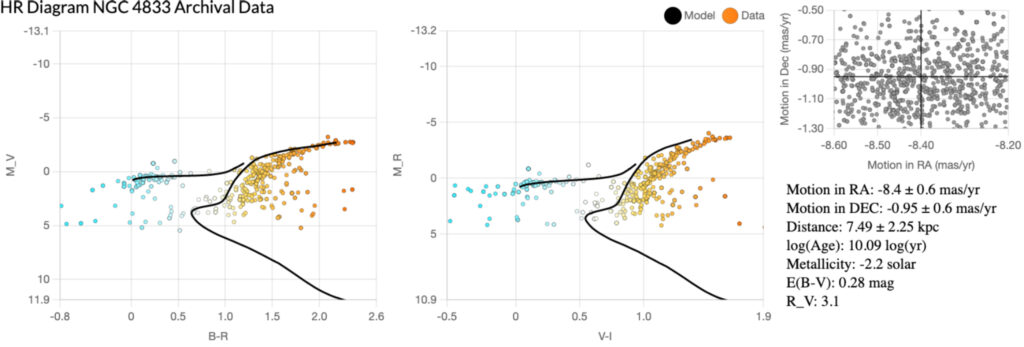
The Data
Our average values for each cluster are described below. Age is measured in log(years), metallicity in solar metallicities (how much more metal there is in each star on average compared to our sun), and reddening in magnitude (how much dimmer it looks from Earth than it would look without any dust in the way).
Cluster | Type | Age (log(yr)) | Metallicity (solar metallicities) | Reddening (magnitude) |
NGC 3293 | Young | 7.13 ± 0.13 | -0.15 ± 0.17 | 0.29 ± 0.04 |
NGC 3330 | Intermediate | 7.67 ± 0.14 | -0.59 ± 0.24 | -1.91 ± 0.39 |
NGC 4833 | Globular | 10.1 ± 0.07 | 0.18 ± 0.04 | 0.26 ± 0.03 |
Analysis
In the young cluster, there are many more hot and bright stars compared to the other clusters: this is indicative of a lot of young massive stars. These stars are often blue in appearance as suggested by the diagram, and as much is evident in the image. As well, there are some redder—or at least less blue—stars in the cluster, which are likely young Sun-like stars. Massive stars burn brighter than Sun-like stars because they exhaust their fuel much more quickly than Sun-like stars, and since they use fuel more quickly, they appear bluer and have hotter surface temperatures, just like smaller flames. As such, it makes sense that a cluster roughly ten to seventeen million (107.13 ± 0.13) years old would look this way: the massive stars are quickly expending their supply of hydrogen in their youth while the smaller Sun-line stars expend their hydrogen more slowly and thus are smaller.
In the intermediate cluster, we see a much more varied mix of stars with fewer blue ones and comparatively more red ones. As well, the stars are much cooler, which makes sense given the context from the discussion of the young cluster. This suggests that there are either many Sun-like stars in this image, or that many of the massive stars are reaching the limits of their hydrogen supply and must begin fusing helium to keep from collapsing. These helium burning stars become red supergiants that are very big and burn very brightly, but they have reduced surface temperatures compared to when they were burning hydrogen. In the HR diagram, the two stars seemingly departed above the lower part of the isochrone are these supergiants. Being roughly thirty-five to sixty-three million (107.67 ± 0.14) years old, it only makes sense that the stars are getting redder, and that is exactly what we see.
Finally, in the globular cluster, we see many yellow and red stars, but some blue stars still remain. At this point, the Sun-like stars are very mature and very bright, but also very cool (as far as stars go); these red supergiants make up the top right portion of the graph. On the left side of the graph are many stars that were once red giants that have now begun to fuse helium and as such are brighter, hotter, and bluer. With time, these helium fusing stars lose mass and become the white dwarfs visible near the bottom of the diagram. At this point, there aren’t really any more massive stars in the cluster, because the life cycle of massive stars is markedly shorter than that of Sun-like stars, and being that the cluster is ten to thirteen billion (1010.1 ± 0.07) years old, this result isn’t unexpected.
Credits
Thank you to Andreas Buzan for working with me on this data, providing the image of NGC 3293, creating the first HR diagram, and for writing the section on how the HR diagrams were made. Andreas maintains his own blog at tarheels.live/blogandreas/.
As well, thank you to Alyssa Manus for also working with me on this data, providing the image of NGC 3330, creating the second HR diagram, and writing the section on how the images were color calibrated. She also runs her own blog at alyssacmanus.wixsite.com/astrophotography.
And finally, thank you to Ruby McGhee for also also working with me on this data, creating the third HR diagram, and for writing the section on how the images were taken. Her blog can be found at tarheels.live/rubymcghee/.